In a network equipped with a detuned filter, a single-line diagram and an equivalent diagram as shown in Fig. 2, the standard behaviour is that the short-circuit impedance (Xcc) at the capacitor bank - network connection point (PCC) is significantly lower than the impedance at each step of the capacitor bank, so that each harmonic current step's absorption of the harmonic currents flowing through the network should be relatively low compared to the one flowing into the network, as this is the path with the lowest impedance.
But the situation may change in the event of networks where the Xcc value is high, i.e. in networks where the short-circuit power (Scc) at the PCC is low. These types of networks are also known as soft networks.
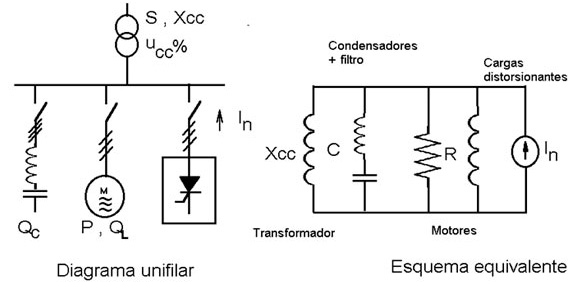
Fig. 2 - Single-line diagram and equivalent diagram of an installation fitted with a detuned filter
Installations that may be vulnerable to these problems are those with low short-circuit power in the High Voltage distribution lines at the low voltage network connection point; or those fed by a power transformer whose K-factor value (harmonic overload factor), by default, is unsuitable for the harmonic contents of the loads it is supplying, or there are long cable sections between the transformer output and the capacitor bank - network PCC, leading to high impedance in this section.
In these cases, the most common effect is an increase in harmonic currents absorbed by the capacitor bank steps. In some cases, such increase may be very serious, severely overloading the capacitors and reactors comprising each detuned filter, and, especially in the case of capacitors, increasing their deterioration, usually in the form of a decrease in capacity. This decrease in capacity even increases harmonic current absorption, because, as can be seen from the formula that determines resonance frequency (Fig. 1), a capacity decrease causes an increase in tuning frequency, meaning it is even closer to the harmonic frequencies in the network (remember that order 5 generally prevails), thereby reducing impedance at that frequency, which therefore increases consumption of currents of that order.
In other words, the detuned filter starts to react in a similar way to a tuned or absorption filter, but, as it is not designed to be used as such, its capacity is exceeded, causing it to deteriorate.
In addition to this effect, the fact that networks with low Scc values, in the event of high harmonic current circulation, usually display high harmonic distortion levels (THD(U)), is another factor leading to an increase in the harmonic current absorbed by capacitors.
In short, any solution that prevents an installed capacitor bank from affecting the network, and, in turn, avoids the capacitor bank itself being affected by the presence of harmonics in the network, may not always solve the problem, with the consequent technical and commercial implications this will undoubtedly entail.